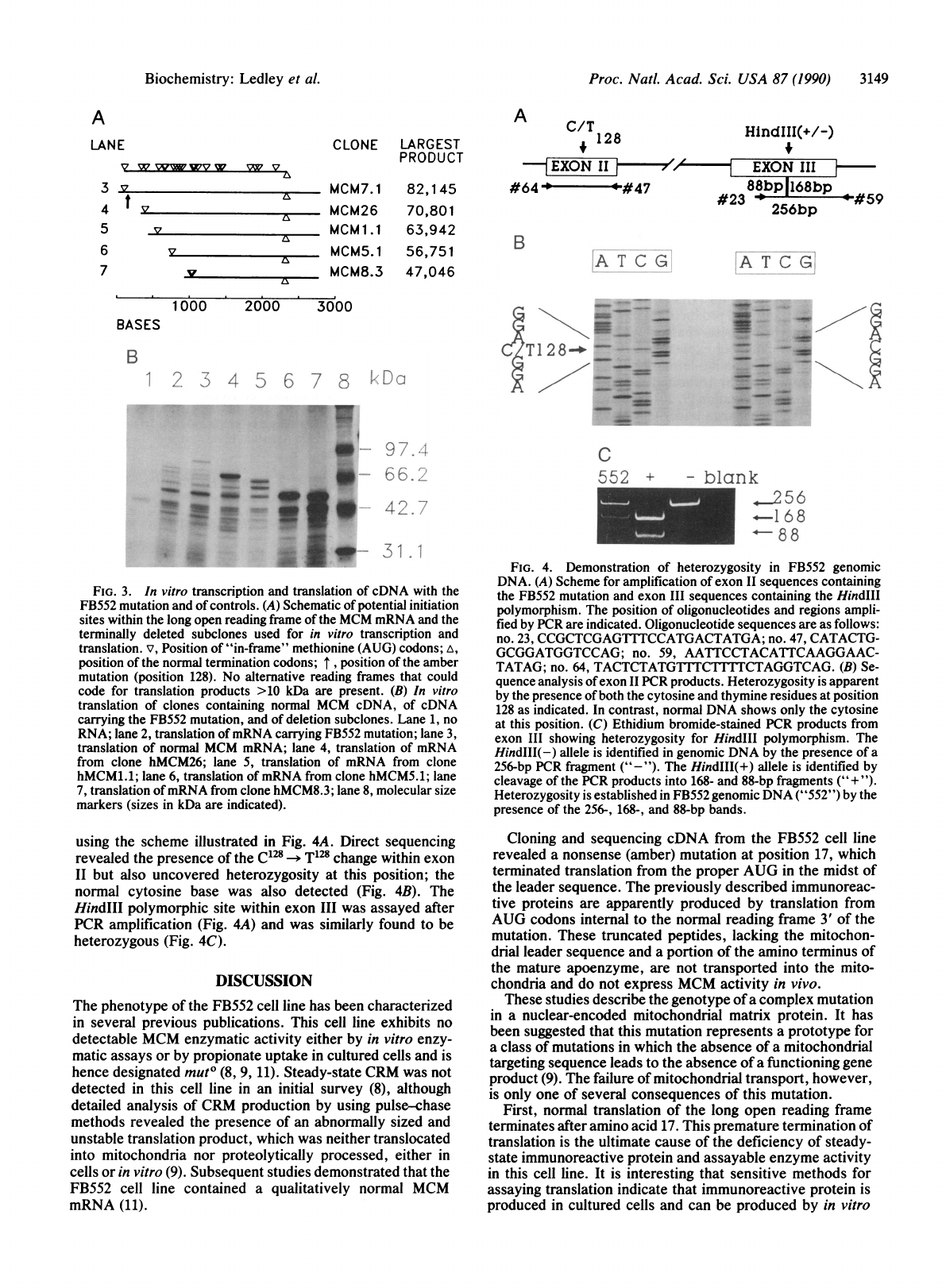
Proc.
Natl.
Acad.
Sci.
USA
87
(1990)
3149
A
LANE
V
w
WWv
wV
w
3
v
4
t
v
5
v
6
v
7
v
CLONE
LARGEST
PRODUCT
MCM7.1
82,145
MCM26
70,801
MCM
1.1
63,942
MCM5.1
56,751
MCM8.3
47,046
A2/
HlndIII(+/-)
t
EXON
II
,'~~N
II
#64
-0
'O
#47
88bp1168bp
#23
4
*
#59
B
A
T
C
G
1000
2000
3000
BASES
B
1
2
3
4
5
6
7
8
..
rs
A
CT
1
2
8
-=
-
-
z
_
t.
RDo
w~~~9
7
_
w
66.3
FIG.
3.
In
vitro
transcription
and
translation
of
cDNA
with
the
FB552
mutation
and
of
controls.
(A)
Schematic
of
potential
initiation
sites
within
the
long
open
reading
frame
of
the
MCM
mRNA
and
the
terminally
deleted
subclones
used
for
in
vitro
transcription
and
translation.
v,
Position
of
"in-frame"
methionine
(AUG)
codons;
A,
position
of
the
normal
termination
codons;
I
,
position
of
the
amber
mutation
(position
128).
No
alternative
reading
frames
that
could
code
for
translation
products
>10
kDa
are
present.
(B)
In
vitro
translation
of
clones
containing
normal
MCM
cDNA,
of
cDNA
carrying
the
FB552
mutation,
and
of
deletion
subclones.
Lane
1,
no
RNA;
lane
2,
translation
of
mRNA
carrying
FB552
mutation;
lane
3,
translation
of
normal
MCM
mRNA;
lane
4,
translation
of
mRNA
from
clone
hMCM26;
lane
5,
translation
of
mRNA
from
clone
hMCM1.1;
lane
6,
translation
of
mRNA
from
clone
hMCM5.1;
lane
7,
translation
of
mRNA
from
clone
hMCM8.3;
lane
8,
molecular
size
markers
(sizes
in
kDa
are
indicated).
using
the
scheme
illustrated
in
Fig.
4A.
Direct
sequencing
revealed
the
presence
of
the
C128
-*
T128
change
within
exon
II
but
also
uncovered
heterozygosity
at
this
position;
the
normal
cytosine
base
was
also
detected
(Fig.
4B).
The
HindIII
polymorphic
site
within
exon
III
was
assayed
after
PCR
amplification
(Fig.
4A)
and
was
similarly
found
to
be
heterozygous
(Fig.
4C).
DISCUSSION
The
phenotype
of
the
FB552
cell
line
has
been
characterized
in
several
previous
publications.
This
cell
line
exhibits
no
detectable
MCM
enzymatic
activity
either
by
in
vitro
enzy-
matic
assays
or
by
propionate
uptake
in
cultured
cells
and
is
hence
designated
mut0
(8,
9,
11).
Steady-state
CRM
was
not
detected
in
this
cell
line
in
an
initial
survey
(8),
although
detailed
analysis
of
CRM
production
by
using
pulse-chase
methods
revealed
the
presence
of
an
abnormally
sized
and
unstable
translation
product,
which
was
neither
translocated
into
mitochondria
nor
proteolytically
processed,
either
in
cells
or
in
vitro
(9).
Subsequent
studies
demonstrated
that
the
FB552
cell
line
contained
a
qualitatively
normal
MCM
mRNA
(11).
C
k
.
56
--1
68
88
FIG.
4.
Demonstration
of
heterozygosity
in
FB552
genomic
DNA.
(A)
Scheme
for
amplification
of
exon
II
sequences
containing
the
FB552
mutation
and
exon
III
sequences
containing
the
Hind1II
polymorphism.
The
position
of
oligonucleotides
and
regions
ampli-
fied
by
PCR
are
indicated.
Oligonucleotide
sequences
are
as
follows:
no.
23,
CCGCTCGAG1TTCCATGACTATGA;
no.
47,
CATACTG-
GCGGATGGTCCAG;
no.
59,
AATTCCTACATTCAAGGAAC-
TATAG;
no.
64,
TACTCTATGTLTCTITTCTAGGTCAG.
(B)
Se-
quence
analysis
of
exon
II
PCR
products.
Heterozygosity
is
apparent
by
the
presence
of
both
the
cytosine
and
thymine
residues
at
position
128
as
indicated.
In
contrast,
normal
DNA
shows
only
the
cytosine
at
this
position.
(C)
Ethidium
bromide-stained
PCR
products
from
exon
III
showing
heterozygosity
for
HindII1
polymorphism.
The
HindIII(-)
allele
is
identified
in
genomic
DNA
by
the
presence
of
a
256-bp
PCR
fragment
("-").
The
HindIII(+)
allele
is
identified
by
cleavage
of
the
PCR
products
into
168-
and
88-bp
fragments
("+
").
Heterozygosity
is
established
in
FB552
genomic
DNA
("552")
by
the
presence
of
the
256-,
168-,
and
88-bp
bands.
Cloning
and
sequencing
cDNA
from
the
FB552
cell
line
revealed
a
nonsense
(amber)
mutation
at
position
17,
which
terminated
translation
from
the
proper
AUG
in
the
midst
of
the
leader
sequence.
The
previously
described
immunoreac-
tive
proteins
are
apparently
produced
by
translation
from
AUG
codons
internal
to
the
normal
reading
frame
3'
of
the
mutation.
These
truncated
peptides,
lacking
the
mitochon-
drial
leader
sequence
and
a
portion
of
the
amino
terminus
of
the
mature
apoenzyme,
are
not
transported
into
the
mito-
chondria
and
do
not
express
MCM
activity
in
vivo.
These
studies
describe
the
genotype
of
a
complex
mutation
in
a
nuclear-encoded
mitochondrial
matrix
protein.
It
has
been
suggested
that
this
mutation
represents
a
prototype
for
a
class
of
mutations
in
which
the
absence
of
a
mitochondrial
targeting
sequence
leads
to
the
absence
of
a
functioning
gene
product
(9).
The
failure
of
mitochondrial
transport,
however,
is
only
one
of
several
consequences
of
this
mutation.
First,
normal
translation
of
the
long
open
reading
frame
terminates
after
amino
acid
17.
This
premature
termination
of
translation
is
the
ultimate
cause
of
the
deficiency
of
steady-
state
immunoreactive
protein
and
assayable
enzyme
activity
in
this
cell
line.
It
is
interesting
that
sensitive
methods
for
assaying
translation
indicate
that
immunoreactive
protein
is
produced
in
cultured
cells
and
can
be
produced
by
in
vitro
-
WN
.Z
-"W
--*W
Biochemistry:
Ledley
et
al.